Next-generation Algorithms for Next-generation Seismology
High-quality seismic data is essential for the detection and characterization of earthquakes. However, such data can also play a useful role in understanding the impacts of hydrological fracking, volcanic eruptions, glacial melt, and other phenomena. In addition to various types of seismographs, researchers have begun to use fiber-optic cables to increase the coverage area of seismic detection — particularly in locations where other types of detectors would be difficult to deploy.
“We want to incorporate fiber-optic data with conventional seismometer data in earthquake early warning systems [and] for monitoring seismicity,” Thomas Hudson, a seismologist at ETH Zürich in Switzerland, said. “It’s a pretty exciting technology from a seismology point of view because you can turn fiber-optic cables into seismometers. There are fiber-optic cables everywhere.”
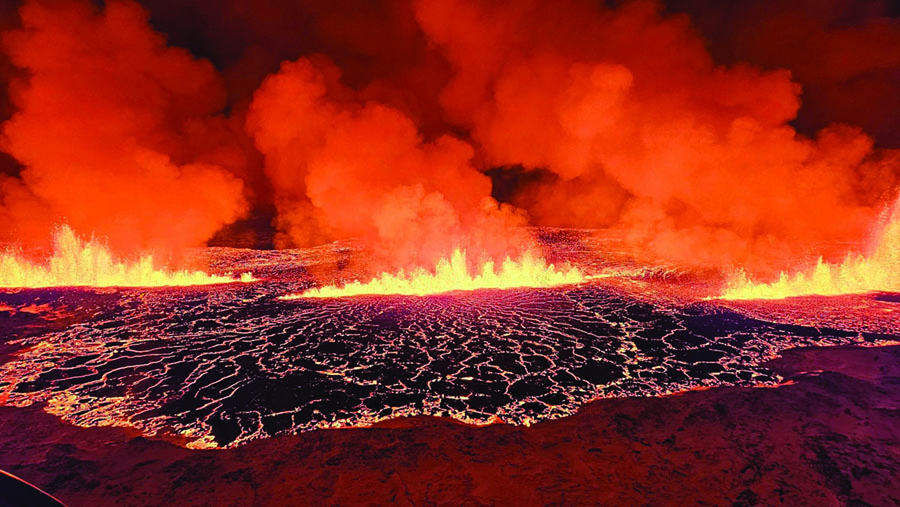
However, analyzing data from arbitrary fiber configurations and using it in tandem with traditional seismometer data is undoubtedly difficult. Most current methods are specific to particular cable geometries, which reduces the flexibility and efficiency of the resulting analyses. For this reason, Hudson and his colleagues have developed a preliminary toolkit that can accommodate arbitrary networks of optical fibers and heterogeneous systems with both fiber optics and traditional seismometers [2]. Their uncomplicated methodology is implementable in a variety of circumstances, with room for further refinements as necessary. The team also demonstrated the algorithm’s utility with real-world data from the Gornergletscher (Gorner Glacier) in the Swiss Alps, the 2023 Svartsengi volcanic eruption in Iceland (see Figure 1), and a geothermal energy borehole in Utah.
“Our paper outlines how we detect any seismic source using fiber-optic data,” Hudson said [2]. “I wanted to provide an algorithm that people could just use out of the box, so that as many people in the community as possible can benefit and try it out. The algorithm is basically a solution to the problem, but I’m not saying that it’s the perfect solution.”
Fiber-optic Seismology
Fiber optics are long, flexible cables that find applications in communication, medical settings, and power transmission. The cables—which are comprised of nonconductive materials—consist of a glass or plastic core that is transparent to visible light and infrared wavelengths, surrounded by a layer of cladding material with a higher refractive index that prevents light from escaping. Light that travels down the core is thus guided through the fiber by a process called total internal reflection. While fiber-optic cables transmit light quite efficiently, the core is solid rather than a vacuum, which means that some of the light is scattered by small-scale density inhomogeneities in the material.1
Distributed acoustic sensing (DAS) exploits this effect. Researchers can measure minute changes in an optical fiber by sending two infrared laser pulses of slightly different wavelengths down the fiber, then comparing the phase difference between the emitted light and the scattered light that returns [3]. During an earthquake or other seismic event, vibrations stretch and compress the fiber, which in turn affects the scattering measurements.
The phase difference is linearly dependent on the strain in the fiber:
\[\Delta \Phi=\frac{4\pi n x_g\psi}{\lambda}\epsilon_{xx}(t,\mathbf{x}),\]
where
\[\epsilon_{xx}=[\boldsymbol{\epsilon}]_{xx}=\bigg[\frac{\partial}{\partial \mathbf{X}}(\mathbf{x}-\mathbf{X})\bigg]_{xx}\]
is the longitudinal component of the strain tensor. The index of refraction \(n\) and Pockels coefficient \(\psi\) are both measurable physical properties of the fiber material. The wavelength \(\lambda\) is determined by the beat frequency between the two pulses of light, and \(x_g \sim 1\) is the gauge length: effectively the sampling distance between two “detectors,” as though the cable is an (imaginary) set of discrete detectors.
DAS can provide meter-scale seismic detections that allow researchers to characterize small earthquakes and other tremors in places that cannot accommodate ordinary seismometers. Since fiber-optic cables are not sensitive to extreme temperature or electrical interference, they are useable in volcanic regions or across glaciers. And while the barriers to seismometer placement are sometimes logistical rather than geological, optical fibers might already be present in certain regions of interest.
“It’s really hard to put seismometers in urban settings because you have to talk to individual landowners,” Hudson said. “Whereas if you want to instrument an entire city [with DAS], you just have to talk to a telecoms company — theoretically. We’re a little way from being able to instrument a whole city.”
The Frontier Observatory for Research in Geothermal Energy in Utah permanently installed a fiber-optic cable on the side of a 1.2-kilometer-deep borehole, which allowed Hudson and his collaborators to perform tests without building any new infrastructure. To analyze the 2023 Svartsengi eruption—for which placing seismometers in the field would have been too hazardous—the team used data from a geothermal energy company’s existing optical fiber that ran alongside a nearby road.
Cover the Earth (With Seismic Detectors)
Despite their promise, fiber optics are not a replacement for other types of seismometers. While DAS can measure strain in one dimension along the length of an optical fiber, modern seismometers measure velocities and/or accelerations in three dimensions (in physics terms, strain is the spatial derivative of the displacement from a seismic event, and velocity is a time derivative of that displacement). In addition to distinctions in data type, these sources produce strikingly different amounts of data. For instance, a single kilometer-long fiber provides 1,000 data channels, whereas researchers might only be able to deploy roughly five seismometers in a given region. The measurements are therefore complementary in many ways, as each has its own strengths.
Hudson and his colleagues addressed the processing of signals from arbitrary fiber configurations, as well as from networks that include both DAS and ordinary seismometers. Each detection method experiences environmental noise, but DAS creates much more instrumental noise than seismometers simply due to the associated physics. As such, scientists must juggle varying signal-to-noise ratios between different components of the heterogeneous network.
“Fiber-optic data is quite noisy, but there’s a lot of it,” Hudson said. “Seismometers are more sensitive, but there are fewer of them. Balancing that is really tough, and our algorithm seeks to do so in a physically motivated way. We effectively allow the more sensitive instruments to contribute more.”
The algorithm, which is implementable via the open-source software package QuakeMigrate, is triggered when detectors pick up a coherent signal of an earthquake or other seismic event that is sharply peaked in space or time (rather than random vibrations). It uses the phase and amplitude from all data channels—weighted appropriately to balance seismometers with fiber optics, if the system includes both—in a process known as back-migration to track the three-dimensional spatial location of the original event and determine when it began (see Figure 2).
![<strong>Figure 2.</strong> Diagram that demonstrates back-migration of a seismic signal. In this simplified example, an underground source produces a wave that propagates in all directions. After a certain amount of time, a set of seismographs on the Earth’s surface measure the attenuated wave, then reconstruct where the event occurred and how much energy it emitted. Figure courtesy of [2].](/media/pwudxucb/figure2.jpg)
Fire and Ice
Earthquakes and volcanic eruptions are the most urgent seismic events to study in terms of disaster management and preparedness. However, Hudson also recognized the need for better data to fully understand the risks of induced seismicity: smaller quakes that stem from human activity, such as hydraulic fracking to extract oil and natural gas from rock, or certain types of geothermal energy production that require drilling [1].
“If you’re going to do geothermal [energy] at scale, you’ll need your geothermal near cities because you don’t want to transport all of that energy inefficiently,” Hudson said. “As you do that, there will be more conflicts [between] society and energy producers [since] geothermal [has] its own risks of induced seismicity. The more sensors near urban settings, the better we understand the risk and mitigate for it.”
In actuality, Hudson’s primary professional interest is cryoseismology—the study of seismic activity that is generated by moving ice—which can help scientists anticipate potential future sea level rise. As glaciers slide across bedrock, they cause their own earthquakes; measuring the resulting waves is one of the few possible ways to probe the situation beneath the ice. “We can use this seismicity to quantify the friction at the interface [between ice and rock],” Hudson said. “The friction at that interface controls how fast the ice slides into the ocean.”
These wide-ranging applications, which encompass natural and artificial disasters both large and small, have inspired Hudson and his collaborators to keep their approach as general as possible. He hopes that other researchers will be able to extend and improve the algorithm, ultimately finding ways to apply it to a wide range of systems. “I tried to write the paper in a way that formulates the problem, rather than just the algorithm,” Hudson said. “I haven’t taken the step of integrating this algorithm into earthquake early warning systems, for example, but I’ve provided the building blocks for someone else to do that.”
1 In physics terms, this phenomenon is known as Rayleigh scattering. It is the same process that makes the sky appear blue.
References
[1] Biello, D. (2013). Fracking could help geothermal become a power player. Scientific American. Retrieved from https://www.scientificamerican.com/article/fracking-for-renewable-power-geothermal.
[2] Hudson, T.S., Klaasen, S., Fontaine, O., Bacon, C.A., Jónsdóttir, K., & Fichtner, A. (2025). Towards a widely applicable earthquake detection algorithm for fibreoptic and hybrid fibreoptic-seismometer networks. Geophys. J. Int., 240(3), 1965-1985.
[3] Lindsey, N.J., & Martin, E.R. (2021). Fiber-optic seismology. Annu. Rev. Earth Planet. Sci., 49, 309-336.
About the Author
Matthew R. Francis
Science writer
Matthew R. Francis is a physicist, science writer, public speaker, educator, and frequent wearer of jaunty hats. His website is bsky.app/profile/bowlerhatscience.org.
Related Reading
Stay Up-to-Date with Email Alerts
Sign up for our monthly newsletter and emails about other topics of your choosing.