The Generation of Rhythmic Patterns in Stick Insect Locomotion
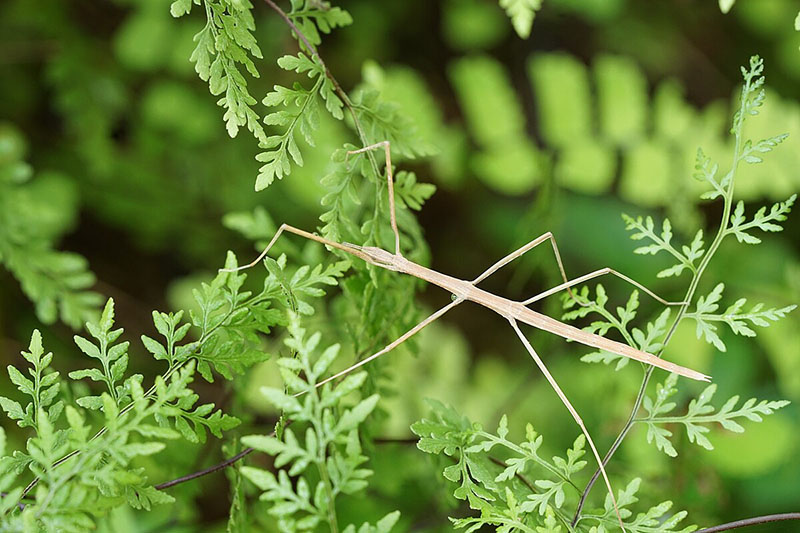
Insects are an advantageous choice for studying the neural circuits that generate complex rhythmic behaviors, given the relative simplicity of their nervous systems yet the intrigue of their stepping patterns. During a minisymposium presentation at the 2025 SIAM Conference on Applications of Dynamical Systems, which is taking place this week in Denver, Colo., Zahra Aminzare of the University of Iowa focused on the generation of movement for a single leg of a stick insect (see Figure 1). She presented joint work with Jonathan Rubin of the University of Pittsburgh, with whom she also co-organized the set of three minisymposium sessions on “Rhythm Generation, Robustness, and Control in Neuronal Networks.”
“Locomotion emerges from complicated interactions between the nervous system, musculoskeletal structures, and factors in the environment,” Aminzare said. “In this talk, I want to focus on the nervous system.” Neural circuits called central pattern generators (CPGs) produce the patterns of neural activity that underly rhythmic motions in animals, like walking or swimming. “As the name implies, these pattern generators don’t need any sensory feedback from the environment or top-down input,” Aminzare continued.
CPGs, which are located in the brain stem, spinal cord, and other structures of the brain, initiate locomotion by signaling to motoneurons that in turn activate the associated muscles. In the mesothoracic leg of a stick insect, three different joints are responsible for different types of movement: vertical motion, lateral motion, and bending (see Figure 2a). Corresponding interneurons and motoneurons—called Ret, Pro, Dep, Lev, Flx, and Ext—send signals to the necessary muscles to ensure that those motions occur (see Figure 2b).
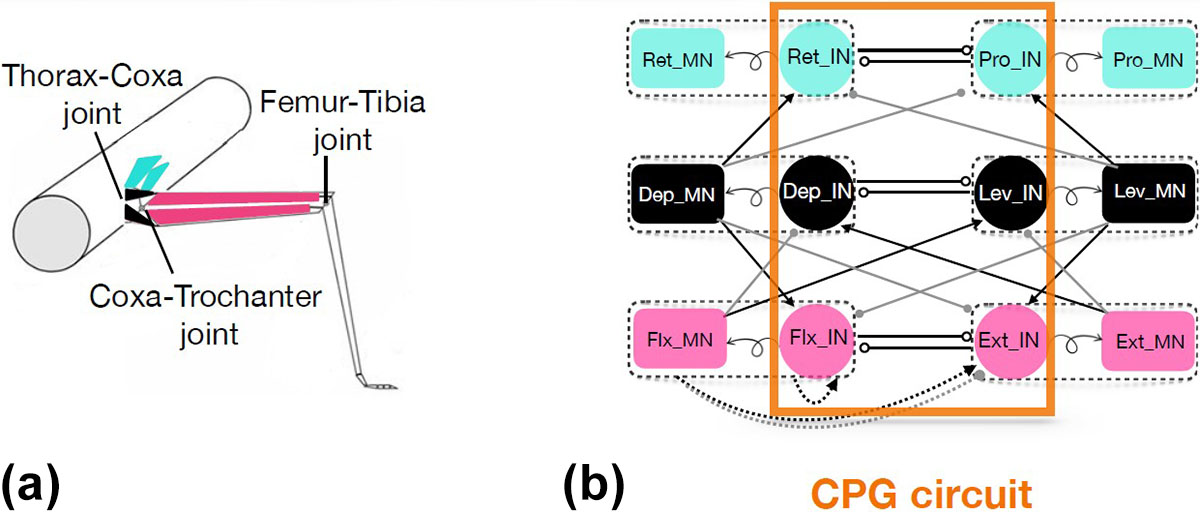
About 40 percent of the step cycle consists of the locomotor swing phase, in which the simultaneous activation of Lev and Ext respectively drives levator and extensor muscles that lift and extend the leg; then, the activation of Pro drives protractor muscles that move it forward. The remaining 60 percent of the cycle duration consists of the stance phase, in which the activation of Dep drives depressor muscles that return the leg to the ground; subsequently, the simultaneous activation of Ret and Flx drives retractor and flexor muscles that move the leg backward and bend it.
Aminzare’s presentation first centered on determining the ideal rhythm for single leg stepping based on experimental knowledge. The rhythm must be periodic in time, and the two interneuron units in each joint must take turns being active; e.g., Ret (which moves the leg backwards) must be silent when Pro (which moves the leg forward) is active. Furthermore, the activation onsets of certain interneurons must be sufficiently synchronized or otherwise timed appropriately. Figure 3 depicts the ideal, theoretical rhythm that is expected when considering CPGs without the presence of sensory feedback.
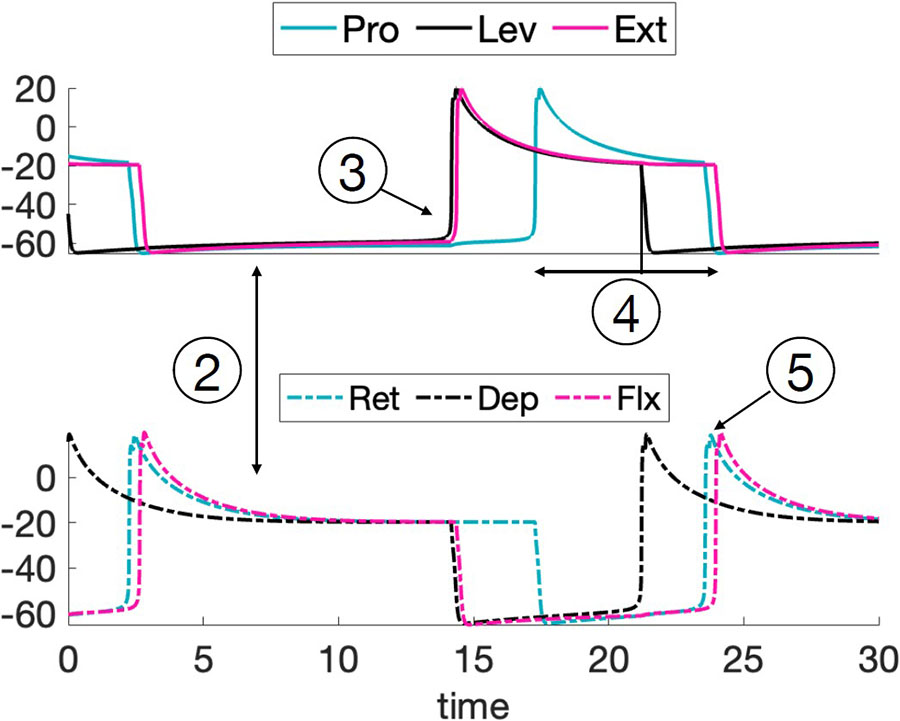
Aminzare then delved into a minimal mathematical model for the CPGs that generate rhythmic motion. The model includes 12-dimensional ordinary differential equations, 23 fixed parameters, and 20 additional tuning parameters to adjust in order to find the right rhythm. Starting with six heterogenous fast-slow systems, she then added six inhibitory synaptic couplings and saw the expected interplay between the activation and silencing of interneurons. In the presence of inhibition, the initiation of oscillations requires the addition of eight excitatory couplings between different interneurons. Ultimately, the rhythm that is generated by this mathematical model appears quite similar to the ideal rhythm in Figure 3.
Three mechanisms in particular enable the excitation-induced oscillations: (i) fast threshold modulation (FTM) for synchronization, (ii) early excitation for small phase delays, and (iii) ghost of saddle node for large phase delays. “How do we organize these three different mechanisms?” Aminzare said. All three must work in concert to ensure that the timing is correct, and she used the clearance time curve to determine the transitions between them.
“We found some relationships between robustness and these mechanisms,” Aminzare continued. The model includes numerous parameters, some of which cannot be changed to any significant degree without causing major issues. However, to maintain robustness, she found that it often worked best to change a group of parameters at a time rather than one single parameter. Out of the excitation-induced oscillation mechanisms, FTM was the most robust. Furthermore, Aminzare also found another group of parameters that controls speed modulation.
Overall, these efforts provided deeper insights into stick insect locomotion and rhythm-generating neuronal networks more broadly. In the future, Aminzare aims to look at the role of musculoskeletal systems and environmental factors in locomotion through the integration of sensory feedback, as well as understand the coordination between different legs of the stick insect.
About the Author
Jillian Kunze
Associate editor, SIAM News
Jillian Kunze is the associate editor of SIAM News.
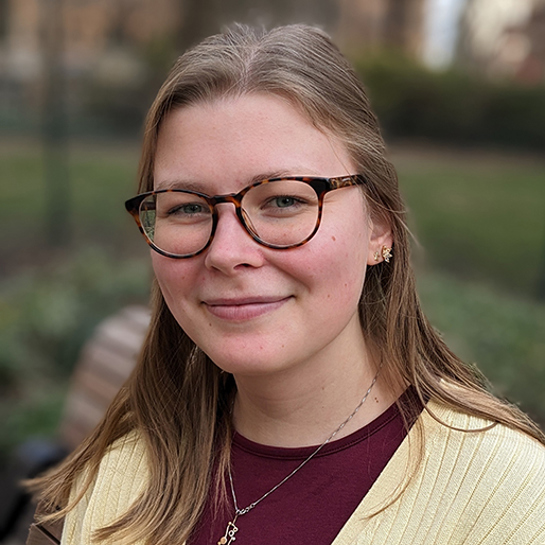
Related Reading
Stay Up-to-Date with Email Alerts
Sign up for our monthly newsletter and emails about other topics of your choosing.